7 RESEARCH AND METHODS
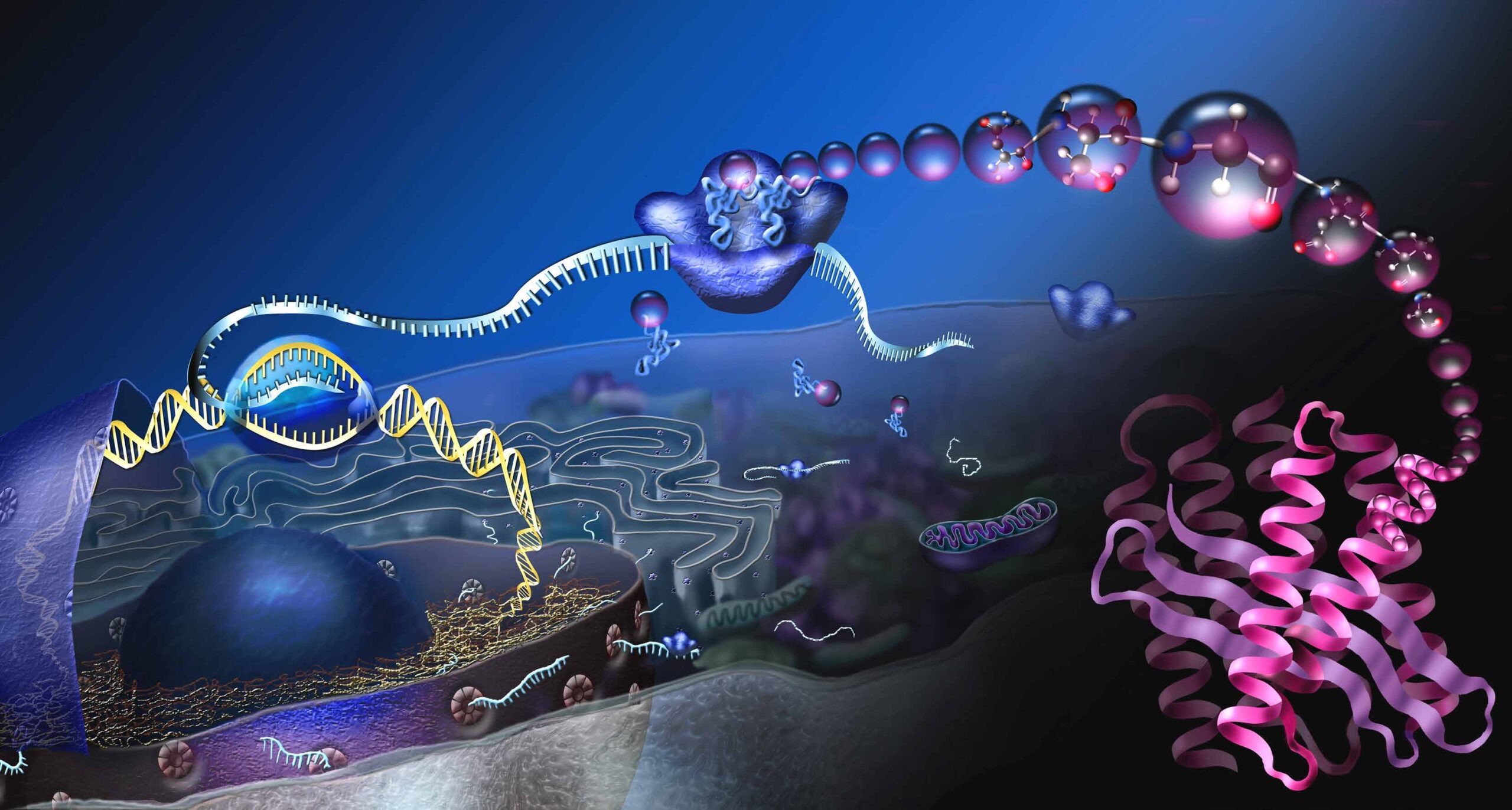
FROM GENE TO PROTEIN
FROM GENE TO PROTEIN
The cell (lat. cellula: small chamber) is the smallest unit of life. It can divide and multiply, has a metabolism and energy turnover, and can grow. Cells can perceive stimuli from the surrounding environment and adapt to it.
The diagram shows a so-called eukaryotic cell and the process of protein production. The cell nucleus A contains the genetic information, also called deoxyribonucleic acid (DNA). The DNA stores the structure of our proteins. For the production of a certain protein, a section on the DNA, a gene, is read B. The transcript is called messenger RNA (mRNA) and enters the cell plasma through nuclear pores C. At the protein factories, the ribosomes, the mRNA is translated into proteins D. Three basic building blocks of mRNA encode a specific amino acid. So-called transport RNAs deliver the amino acids E, the basic building blocks of proteins. At the ribosomes, the amino acids are linked together and long protein chains are formed F. In order for the protein chains to become functional molecules, they must fold into specific, three-dimensional shapes G.
ANALYTICAL METHODS
ANALYTICAL METHODS
To study biochemical processes and macromolecules, i. e. proteins, DNA, RNA or carbohydrates, our researchers use methods and techniques from classical chemistry, physics, molecular biology, microbiology, biotechnology and also computer science on a routine basis. They are interested in the structure of molecules, how they function and how they interact at the molecular or cellular level, and also in understanding
their role in an organism. Depending on the analytical method, biomolecules, such as proteins, are first extracted from cells. Using various separation techniques such as centrifugation, gel electrophoresis or chromatography, cell lysates can then be separated according to their properties, such as size or charge. To make certain molecules visible in the microscope, they can be labeled in various ways and certain structures can be stained. Genetic engineering enables the targeted production or manipulation of proteins.
For successful research, the method services, provided by so-called facilities is an important pillar of the institute. These central facilities support all departments with their specialist expertise by providing a chemistry service, performing DNA sequencing, microscopy service and protein production as well as analysis service.
METHOD DEVELOPMENT
METHOD DEVELOPMENT
In order to expand the frontiers of knowledge, even basic research always involves improving current methods and technologies and developing new ones. From the very beginning, some departments at the institute focused on method development: Kurt Hannig, for example, headed the department of biochemical working methods. His team developed a separation method for cells and cell organelles. Pehr Edmann became known for his protein sequencing method.
Wolfram Zillig is considered a father of molecular genetics. His team developed the “phenol method” for purifying nucleic acids, which still is part of standard practice around the world. They also established for the first time in vitro a protein biosynthesis system of the bacterium Escherischia coli. Peter Hans Hofschneider, a pioneer in molecular biology, investigated bacteriophages— viruses that infect bacteria. Together with other research groups, the phage M13 (Munich 13) was used as one of the first cloning vectors. Carriers, lat. vectors, are used in genetic engineering to transfer foreign genetic information into a living recipient cell.
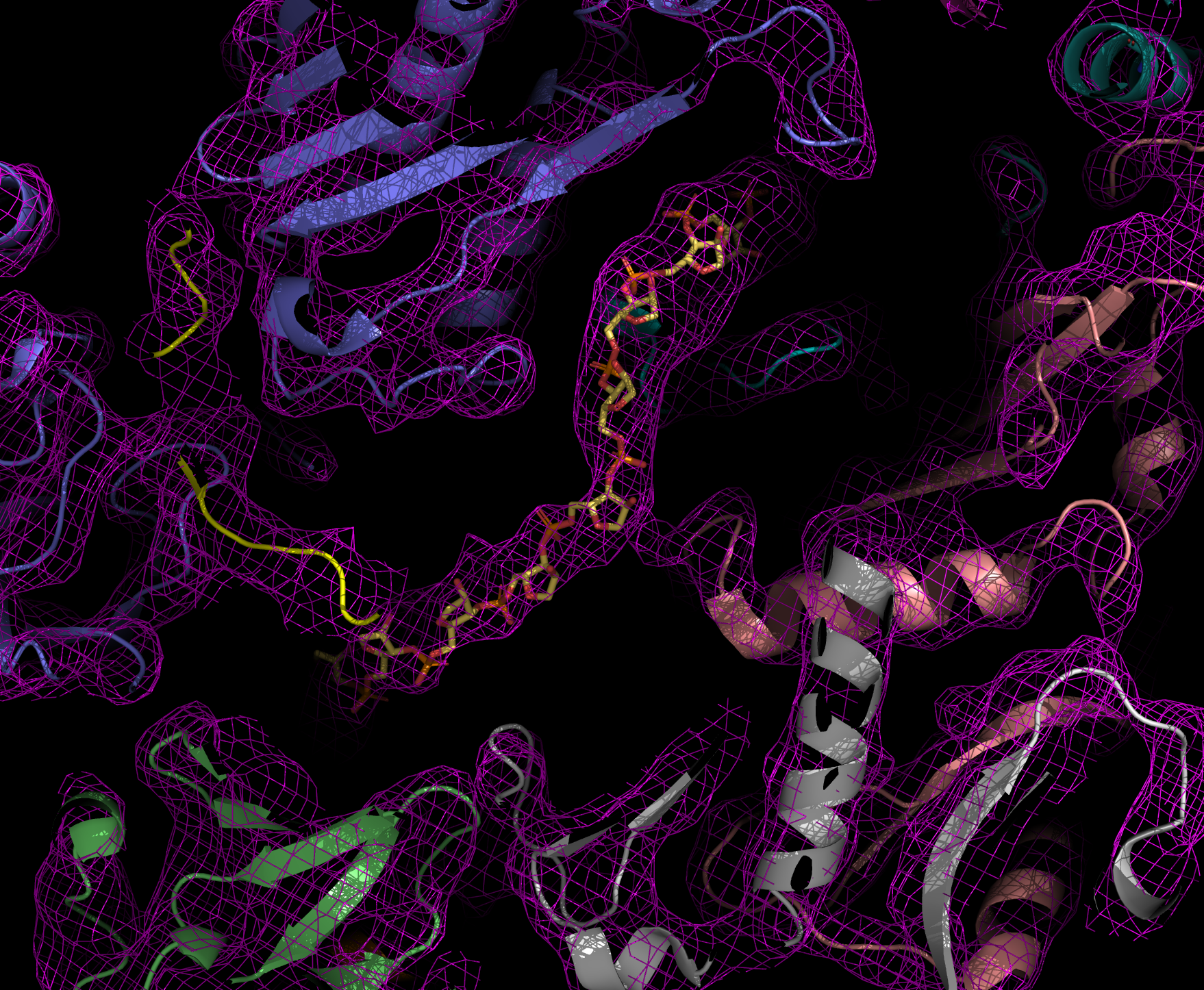
ANALYSIS OF PROTEIN STRUCTURE
ANALYSIS OF PROTEIN STRUCTURE
Walter Hoppe, former director of the Structural Research Department, was one of the pioneers of X-ray crystallography in Germany. Later, however, he also developed visions of how electron microscopy could be used for the three-dimensional structural analysis of proteins.
X-ray structural analysis is used to determine the atomic structure of a protein crystal. To crystallize, proteins must be very pure and arrange themselves regularly. When X-rays are shone through the crystals, the beams are diffracted. The diffraction pattern allows conclusions to be drawn about the structure of the proteins.
Thanks to this method, Robert Huber, Hartmut Michel and Johann Deisenhofer succeeded in elucidating the three-dimensional structure of the reaction center of photosynthesis in a bacterium, which was later honored with the Nobel Prize. The Department of Cellular Structural Biology, led by Elena Conti, also uses protein structural analysis to understand the structure and function of the exosome and its interaction partners. The exosome is a molecular machine that degrades RNA molecules in cells.
CRYO-ELECTRON MICROSCOPY
CRYO-ELECTRON MICROSCOPY
In contrast to X-ray structure analysis, cryo-electron microscopy (cryo-EM) does not require the preparation of protein crystals. Many proteins are very unstable or do not form crystals. The proteins remain in their natural form. In recent years single particle analysis has developed into an efficient method for high-resolution protein structure analysis. It is particularly suitable for large molecular complexes, such as the 26S proteasome, a huge complex composed of 34 different subunits, which is responsible for the targeted degradation of proteins that are no longer needed.
For cryo-EM, proteins are snap-frozen to below -150°C. This freezes the water in the samples to a glass-like state without ice crystals. The samples are then imaged with an electron beam. With the help of computers, high-resolution 3D images are calculated from many different snapshots of individual proteins.
Wolfgang Baumeister is considered the pioneer of cryo-electron tomography, which can be used to study the molecular architecture of cells at high resolution (< 1nm). John Briggs’ department uses this method to study enveloped viruses.
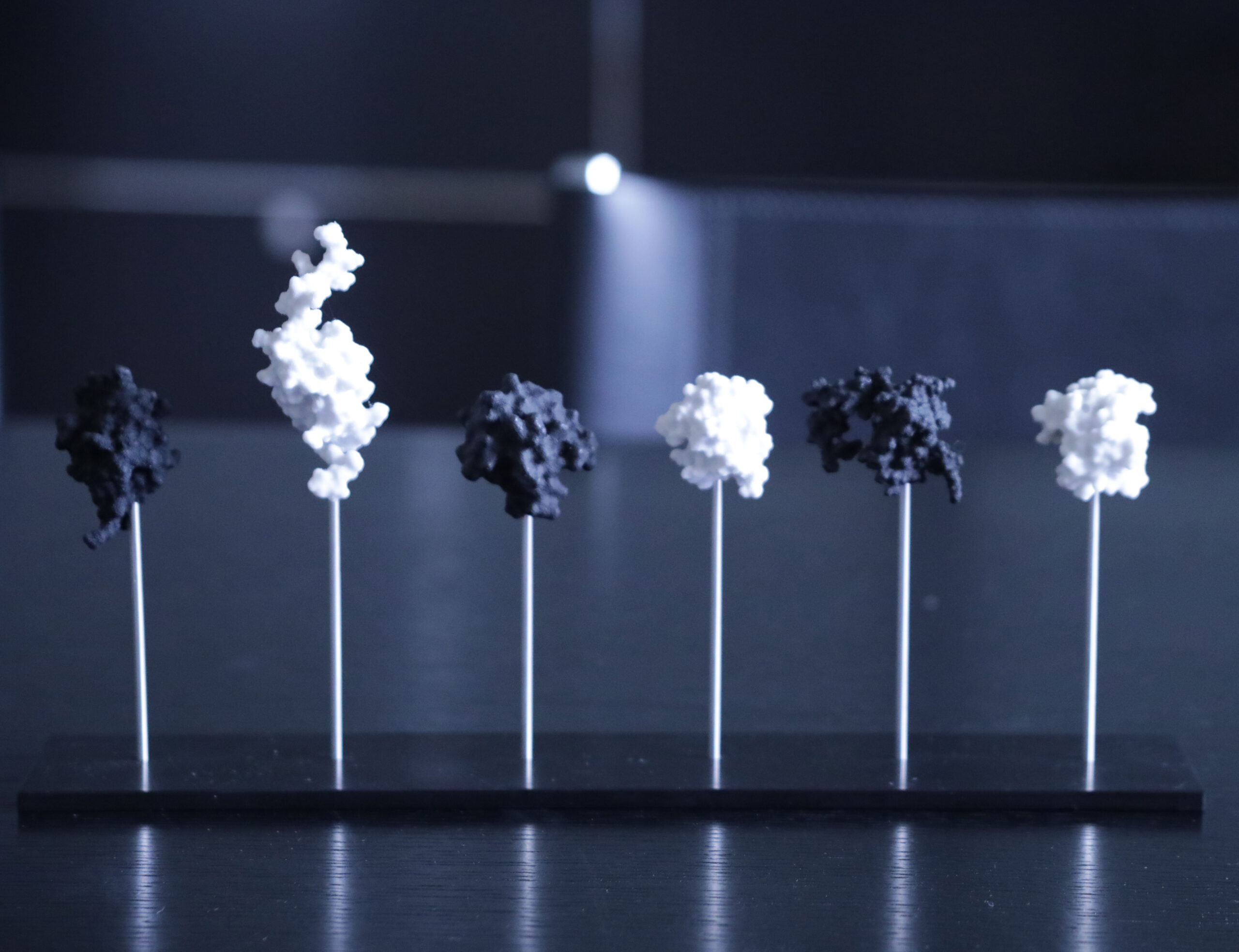
UBIQUITINS—SMALL PROTEINS WITH A BIG EFFECT
UBIQUITINS—SMALL PROTEINS WITH A BIG EFFECT
Ubiquitin is a small protein that tags other proteins to direct their downstream fates. It had been known for years that one type of ubiquitin tagging directs to the “protein shredder”, the proteasome, where they are degraded. Stefan Jentsch discovered that ubiquitin tagging also serves other important purposes, such as to orchestrate DNA repair.
Today we know that ubiquitins and several ubiquitin-like proteins (UBLs) control timing, subcellular location, composition, conformation, and activities of thousands of dif- ferent proteins and macromolecules in cells. Defects in ubiquitin and UBL response pathways lead to numerous diseases such as cancer, neurodegenerative disorders, and viral infections.
Brenda Schulman and her team have discovered how ubiquitin and UBL tags are precisely delivered to the right proteins, through large, dynamic molecular assemblies.
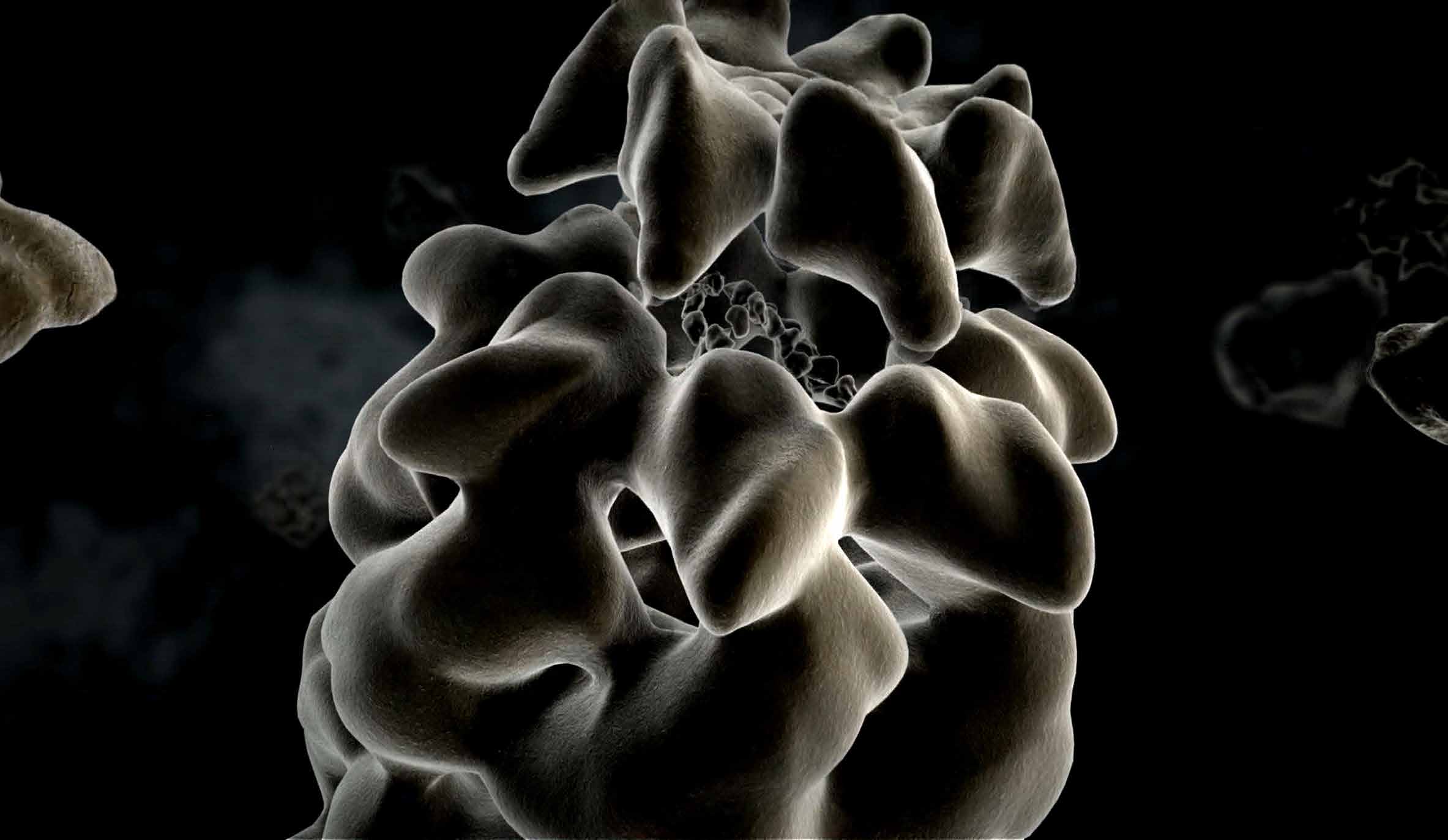
PROTEIN FOLDING
PROTEIN FOLDING
There are numerous ways in which amino acid chains can be folded to form proteins, but only one is the right way to guarantee that a protein fulfills its tasks. “Cellular chaperones” ensure correct protein folding and help to ensure that the correct amino acid strands of a protein that has just been produced find each other—a discovery by F.-Ulrich Hartl and his research focus until today. Chaperones may even be able to correct misfolding that has occurred. If this does not happen, the proteins can clump together, deposit in cells and damage them. Neurodegenerative diseases such as Alzheimer’s, Parkinson’s or Huntington’s chorea can be the result. Research into chaperones is so important for understanding these diseases.
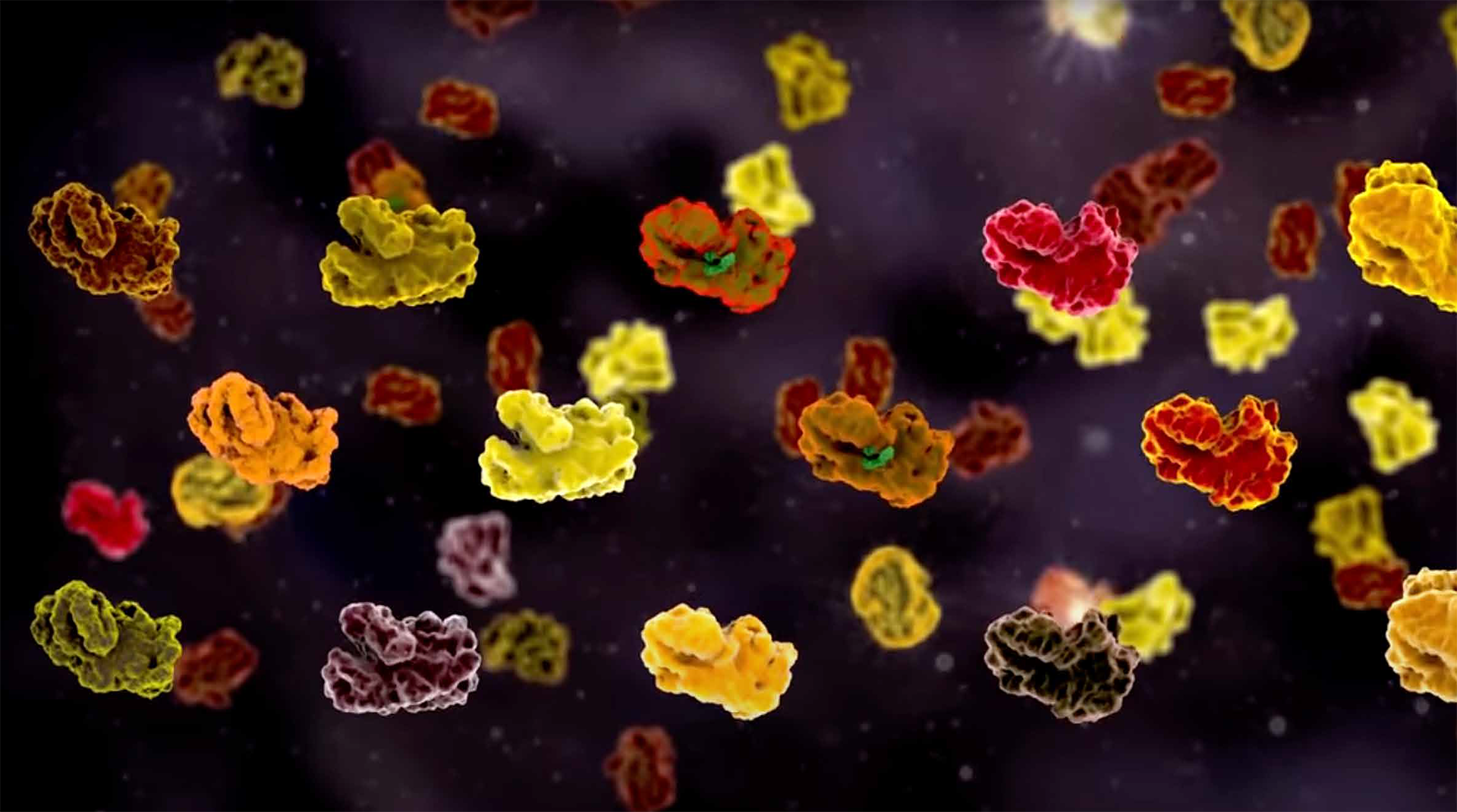
THE PROTEOM
THE PROTEOM
Im Gegensatz zum Genom ist das Proteom eines Organismus – also die Gesamtheit aller Proteine – nicht für alle Zellen gleich: Jeder Zelltyp hat eine spezifische Proteinausstattung. Auch innerhalb der Zelle ist das Proteininventar variabel, denn Proteine werden permanent gebildet, verändert oder entsorgt. Diese dynamischen Prozesse untersuchen Matthias Mann und seine Mitarbeiter*innen mit Hilfe der Massenspektrometrie. Mittlerweile hat das Team die Massenspektrometrie so weit optimiert, dass ihre Nutzung in der medizinischen Diagnostik immer weiter in den Fokus rückt. So können mit dieser Methode die Proteine, z. B. aus einem Blutstropfen, identifiziert und so der allgemeine Gesundheitszustand überprüft werden. Da die Proteomforschung von Matthias Mann so viele Bereiche in der biologischen und medizinischen Fachwelt beeinflusst, ist er mit seinen wissenschaftlichen Veröffentlichungen weltweit einer der meistzitierten Wissenschaftler.
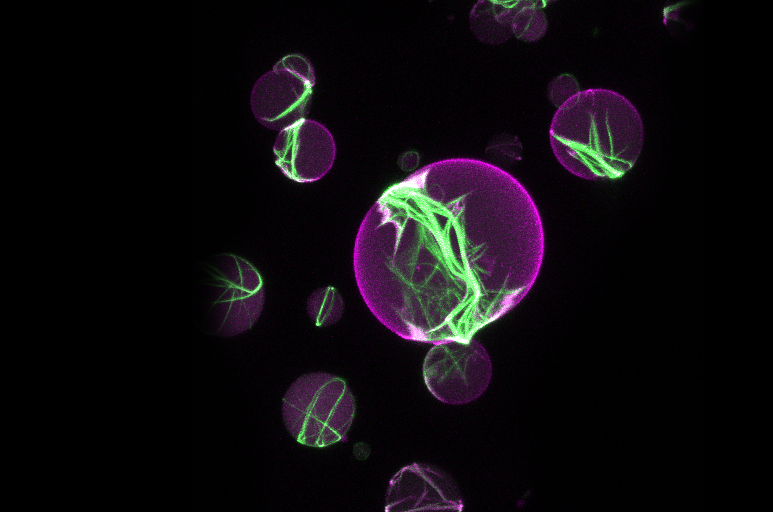
CELL DIVISION
CELL DIVISION
Cell division is a fundamental property of life. As a biophysicist, Petra Schwille uses a synthetic mechanistic approach to investigate which proteins are essential for cell division. The group was able to show that the interaction of just five proteins leads to the formation of a division ring structure in the center of a fat vesicle (liposome) — a possible first step towards replicating the cell division machinery for artificial cells in the test tube.
Erich Nigg researched mitosis, the process of nuclear and cell division. His team investigated which mechanisms regulate mitosis in time and space and how error-free division is ensured. Disturbances in these processes are held responsible for the development of cancer cells.
Axel Ullrich has also studied the development of cancer cells. With his team, he has studied growth factor receptors on cells and thus the signaling pathways that stimu- late cells to divide. The targeted blockade of the overactivated signaling pathways inhibits cell division — the basis for certain cancer drugs.
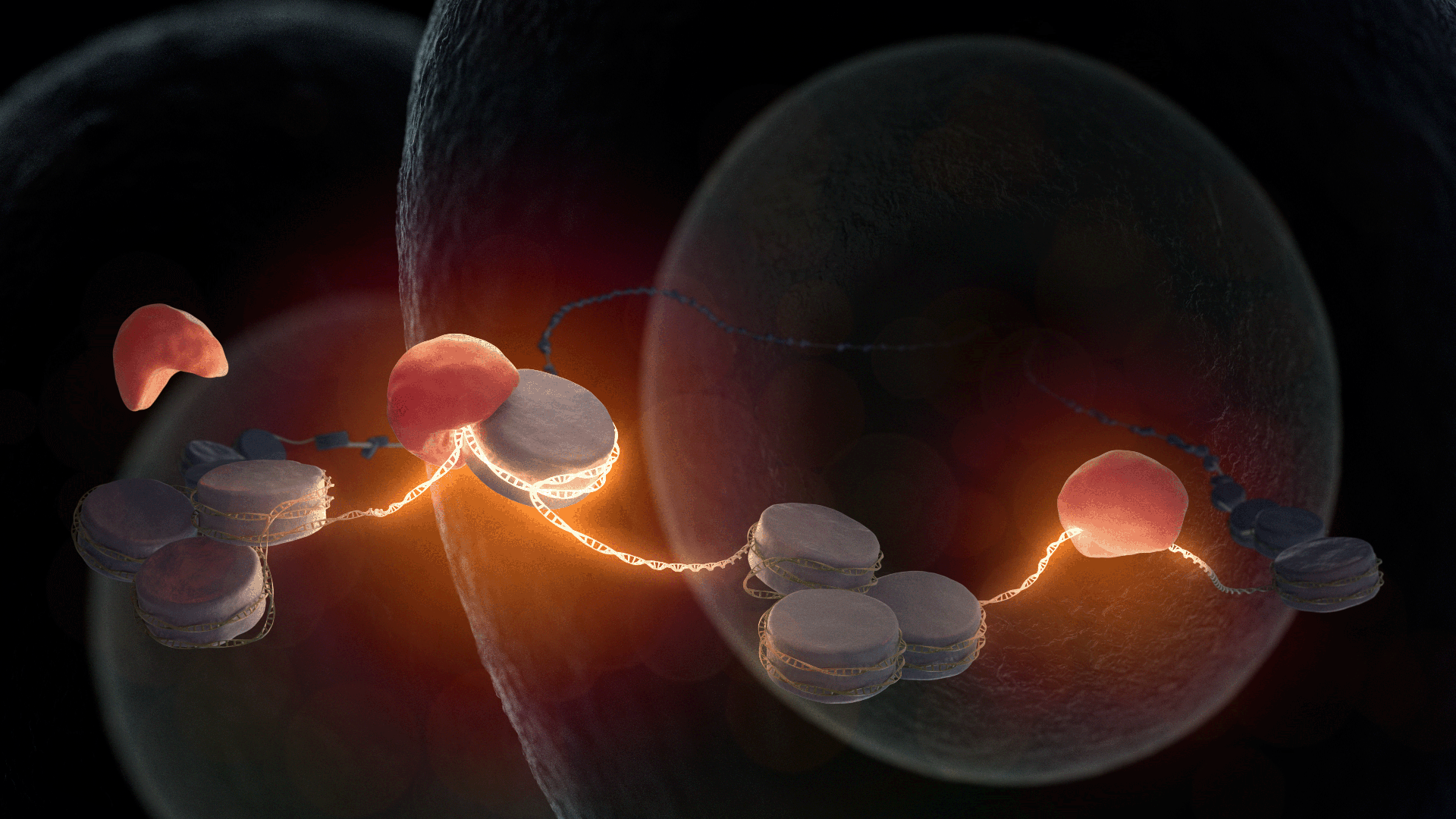
THE BEGINNING OF LIFE
THE BEGINNING OF LIFE
Kikuë Tachibana’s department studies the mechanisms of reprogramming cells to a totipotent state—these cells have the potential to develop into all cell types and an entire organism. Totipotent cells are generated by fertilization of an egg by sperm. The maternal and paternal genetic information is newly combined. After fertilization, the combined genetic information is still inactive in the cell nucleus. While the first division of the fertilized egg cell still functions with the help of the maternal factors stored inside it, the further development of an embryo requires the synthesis of new embryonic products. Kikuë Tachibana’s team has only recently discovered a key factor that is responsible for opening the DNA. Many questions about the molecular activation and restructuring of totipotent cells have yet to be answered. They will occupy the department intensively in the coming years.
THE INTERCELLULAR SPACE
THE INTERCELLULAR SPACE
The extracellular matrix (ECM) is the area between cells. The substances and fibers found in the intercellular spaces hold all tissues together in multicellular organisms. Klaus Kühn is considered a co-founder of connective tissue research. With his group, he deciphered the structure of collagen fibers — an important component of the ECM, contained in skin, tendons, bones and cartilage. Kühn determined the exact sequence of the three intertwined amino acid chains that make up the collagen I protein.
Other important components of ECM are laminins—collagen-like proteins that have binding sites to cell surface receptors. Laminins were isolated and their structure elucidated by Rupert Timpl. He is considered the founder of modern basal lamina research, which has been groundbreaking for developmental biology, as well as the diagnosis and treatment of many diseases.
Reinhard Fässler and his colleagues are investigating the function of integrins— receptor proteins that are embedded in the cell membrane and extend into the ECM. Integrins can trigger biochemical signals and both transmit and receive mechanical signals. Integrins influence cell division or cell migration, which play an important role in blood clotting and immune defense.
ARCHAEA— THE EXTREMES
Starting in the 1970s, Wolfram Zillig was fascinated by the newly discovered primordial realm of living organisms, the archaea. These unicellular organisms form a third type of life alongside bacteria and eukaryotes (organisms with a cell nucleus). They colonize sites with extreme living conditions, such as boiling hot springs or habitats with extremely high salinity. Zillig’s team showed that
a great similarity in gene expression exists between archaea and eukaryotes, while metabolic genes of eukaryotes correspond more to bacterial genes. Thus, he formulated the hypothesis that eukaryotes arose from a fusion of bacteria and archaea.
Dieter Oesterhelt was interested in the salt-loving archaeon Halobacterium salinarium. In the early 1970s, he detected retinal in the cell membrane of the archaebacterium. As a component of the protein rhodopsin, retinal is involved in the visual process in the retina of most vertebrates, including humans.
The newly discovered protein Bacteriorhodopsin is a light-driven proton pump. With the help of this pump, a gradient is created, a proton concentration gradient between the inside and the outside; in this way, an electrical potential is built up across the membrane. The process is similar to charging a battery. Thus, the light absorbed by the retinal is converted into electrochemical energy and used in this form by the archaebacterium. The work of Dieter Oesterhelt and his team on the interaction of light with protein-pigment complexes has laid the foundations for today’s field of optogenetics.
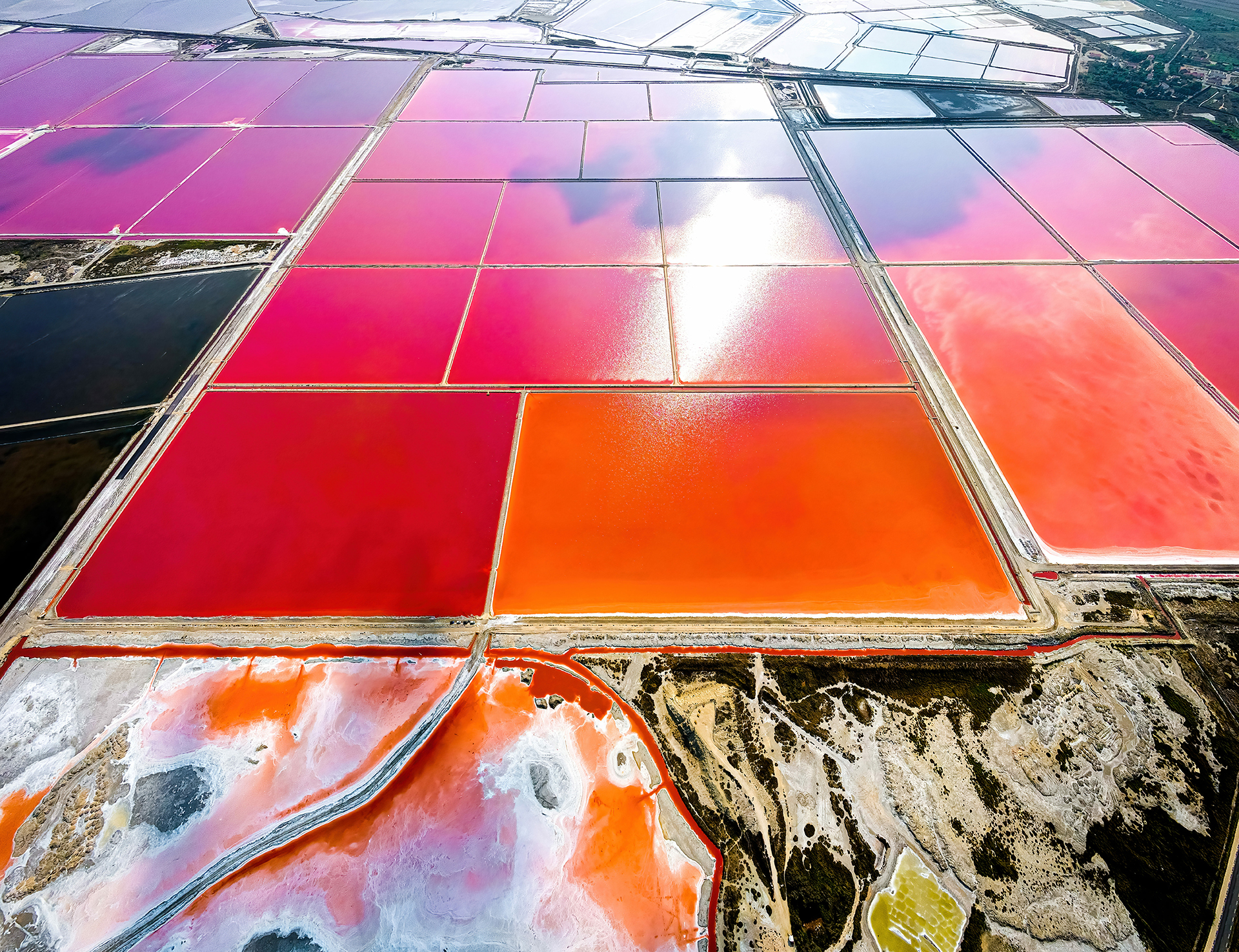
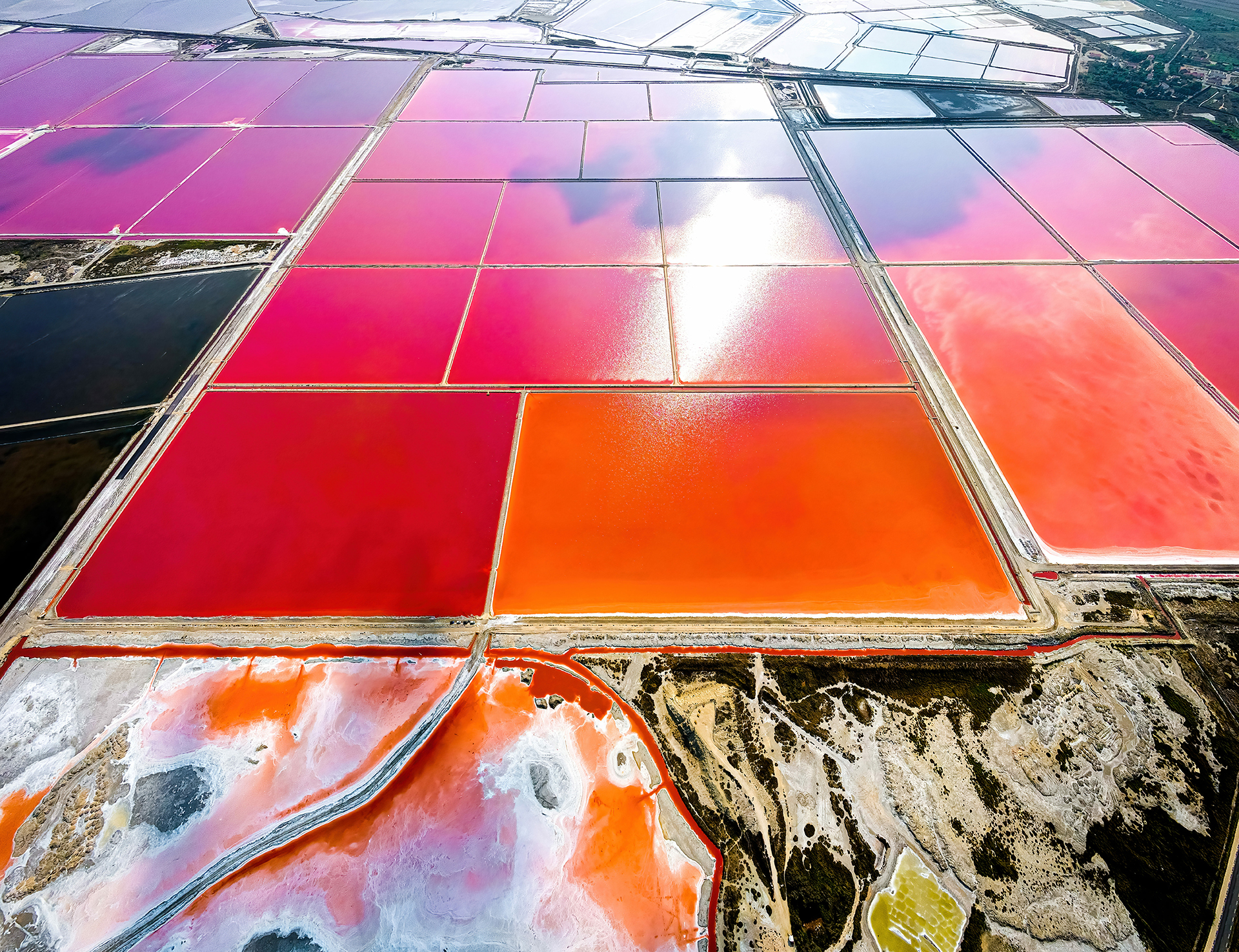